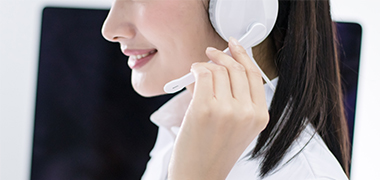
- Contact:Minister Xu
- Mobile:13841408476
- Tel:024-44837288
- Fax:024-44837004
- E-mail:xuming58@126.com
- Web:natashaterry.com
- Address:No.110 XiangHuai Road
Benxi Economic Development Zone
Liaoning Province
Optimal Machine Performance Depends on Bearing Selection, Part 1
05 Nov,2018
How should you select a bearing to meet the various demands of vertical and horizontal CNC machining, to improve both productivity and performance?
It wasn’t so long ago that a typical automotive engine or transmission plant would maintain many machines, each dedicated to a specific and narrow set of demands. Together, this collection of dedicated machines was configured into a transfer line, where a raw workpiece would travel down the line with each machine performing to a narrow set of milling, drilling, or tapping operations — known as a duty cycle — transforming it into a finished part. The spindle bearings in each of those machines were optimized for its specific duty cycle.
Times have changed and so has the typical plant. Transfer lines have been replaced by flexible CNC machining centers to increase efficiency, for smaller volumes and more frequent product changes. CNC machines may range from three- to nine-axes (typically five axes) and use automatic tool changers, magazines, carousels, and robotic workpiece material handling systems to perform multiple operations, driving down cycle time and helping drive efficient operations on plant floors around the world.
But in the world of machining, different tasks have different demands. Many of these different operations and materials have diametrically opposed demands on the milling spindle, and therefore the milling spindle bearing. Materials may range from traditional high-alloy steels and cast iron to aluminum, titanium, and nickel alloys. The material, along with the cutting operation, dictates the loads and speeds that must be applied, with further implications for the bearing.
Few individual components affect overall performance as profoundly as the milling spindle bearing. The bearings define the speed, load carrying capacity, precision and stiffness. The bearings contribute to the spindle operating temperature, vibration and noise during operation. Machine tools must be extremely accurate, reliable, and capable of high levels of productivity. A bearing’s precision level has a major influence on the ability to perform in a production environment. When the bearing stops working as intended, the entire machine goes down for repair.
Optimized performance greatly depends on selecting the right bearing for the right machining application. Whether you’re a machine tool spindle designer at an OEM, or a maintenance, repair and overhaul shop, the demands of the end-use application are critically important to consider when making a bearing selection.
Comparing the demands of different jobs. Within a given machining application, speeds, loads and other factors may vary drastically. Finish cuts are used to generate superior surface finish with minimum chatter, whereas roughing cuts remove the most amount of material in the shortest period of time.
Finishing cuts are shallow and precise with a small tool diameter at a very high spindle speed, with a very low feed-rate (linear speed of the tool through the workpiece.) As a result, they generate very low tool loads but require the spindle to perform well at a very high speed.
Roughing cuts are deep and wide with a large-diameter cutter running at a relatively slow spindle speed at a very high feed-rate, generating a significantly higher amount of load on the tool that is supported by the spindle bearings.
Optimized bearing selection. Each of the different conditions described above makes unique demands on the milling spindle bearing itself. Consider that the needs of a roughing cut versus a finishing cut are opposite, requiring differing respective bearing performance (low speed/high loads versus high speed/low loads.) Here’s why: As speed increases in any application, so does centrifugal force on each of the balls within the spindle bearing.
This centrifugal force acts radially away from the center of the bearing’s rotation (assuming a rotating reference frame), with the outer raceway preventing the balls from being thrown out of the bearing. As centrifugal force increases here, the result is elevated contact stress between the raceway and the balls. Increased centrifugal force also drives the point at which each ball contacts the outer raceway toward the bottom of the raceway.
However, the exact opposite occurs between the ball and the inner raceway, resulting in inner and outer contact angles that are not equal. This phenomenon is referred to as the contact angle shift. Because the ball is a solid body it can have only one axis of rotation and therefore increased contact angle shift results in sliding, greater heat generation, higher operating temperatures, and increased surface wear on the balls.
One method to minimize the impact of the effects of increased centrifugal force is simply to reduce the weight of the balls. There are two ways to accomplish this:
1) Reduce the ball size and/or
2) Convert from steel to ceramic, because ceramic balls are 40% lighter than their steel ball counterparts. The lighter balls generate lower centrifugal force which does not completely offset the higher contact stress resulting from the higher modulus of elasticity of the ceramic. However, the lower centrifugal force reduces the contact angle shift, which results in less heat generation caused by sliding.
A second method is to increase raceway radius. The relationship between the raceway radius and the ball diameter is referred to as the raceway curvature and is mathematically described as the raceway radius divided by the ball diameter, expressed as a percent. For example, if you have a raceway radius of 0.5 mm and a 1 mm diameter ball, the raceway curvature is 50%. A 50% raceway curvature implies line-to-line contact and the bearing would not turn freely, so curvatures are always greater than 50%.
Curvatures below 54% are considered closed while curvatures that are greater are considered open. Bearings with closed curvatures are better at carrying loads but generate more heat, while open bearings can run faster but have reduced capacities.
Using either of these two measures to increase the “speed ability” of the bearing can drastically reduce the bearing’s ability to carry loads and therefore reduce the ability of the spindle to rough cut. Typically, there are two to three designs available for a given ISO dimensional series that incorporate various ball size and curvatures to optimize performance for a particular cutting profile. This is why it is important to consider the intended duty cycle of a machine, so the optimum design and ball material can be selected.
Let’s take a look at some examples:
Roughing and maximum metal removal. Spindles that are designed for heavy tool loads should have bearings with the largest steel balls that will fit into the bearing cross section (> 50% of the bearing section) as well as the closed raceway curvature, to reduce the contact stress between the raceway and ball. The large ball diameter and closed curvature suit the heavy loads and slower speeds associated with roughing, which may range between 500 rpm and 1,500 rpm, depending on the cutter diameter, material removal rate, and workpiece material. Most roughing spindles use grease as the lubrication method and are rated from 3,500 rpm to 7,500 rpm depending on the machine size.
Finishing and maximizing speed. When designing a spindle for higher speeds for dedicated finishing cuts, minimizing heat generation should be the guiding principle and the large ball/closed curvature bearing described above becomes less desirable due to excessive heat generation inherent to the design. Choosing a small ball design (< 50% of the bearing section) with open curvature will yield low heat generation and result in a lower spindle temperature. High-speed finishing spindles operate between 7,500 rpm and 24,000 rpm and use metered air/oil as the lubrication method. Dedicated roughing spindles are typically rated from 15,000 rpm to 30,000 rpm depending on the machine size.
Multipurpose applications, optimized for load and speed. If the design goal is a spindle that can perform both heavy cuts at lower speeds and delicate cuts at high speeds, the selection of a bearing that can handle these opposite needs is imperative. These needs—and everything in between—are common for vertical machining centers. Designers should seek a precision bearing with balanced capabilities that can bridge the gap. A bearing with a medium ball design (~ 50% of the bearing section) with moderately open curvature will yield low heat generation and decent load carrying capacity. Mills that are required to perform both roughing and finishing operations can see speeds as low as 500 rpm and as high as 12,500 rpm, and use either grease or metered air/oil as the lubrication method. Multipurpose spindles are typically rated from 7,500 rpm to 15,000 rpm depending on the machine size.
Ceramic balls. Ceramic balls are commonly chosen when the spindle’s duty cycle is dominated by high-speed finishing operations. In addition to lower density/weight, ceramic balls have a higher modulus of elasticity than their steel ball counterparts, which yields a smaller contact ellipse (the shape of the contact area between the raceway caused by elastic deformation of the ball and rings.) The smaller the contact area, the higher will be the contact stress between the ball and raceway under the same loading. If the external loading does not yield excessive stress between the ball and the raceway, then the bearing will generate less heat due to the smaller contact area.
The bearing configuration that achieves the best balance of operational dynamics across the entire duty cycle for a given application is the optimized bearing solution.
Next … Optimal Machine Performance Depends on Bearing Selection — and Installation
Eric Faust is an application engineering specialist for The Timken Company, and the author of several technical papers on bearing selection. Timken has conducted extensive testing and analysis on its own bearing solutions to help customers make informed choices. The range of options has demonstrated the desired performance for a wide variety of machine tool applications, and specialists are available to assist in bearing selection for OEMs, repair shops or end-users.